Introduction to the Long-Term Evolution Experiment (LTEE)

By Richard E. Lenski
Contents
- The Rhythm of the LTEE
- The Goals of the LTEE
- What We’ve Learned from the LTEE
- How Long Will the LTEE Continue?
The Rhythm of the LTEE
The LTEE is a pretty simple experiment, both conceptually and methodologically. The core idea is to observe and quantify the process of evolution in action. We can do that by taking advantage of the speed with which bacteria reproduce, their large population sizes, the ease with which they can be grown in the lab, and the fact that one can preserve bacteria in suspended animation (frozen) and bring them back to life.
The LTEE has 12 populations of E. coli, all started in 1988 from the same non-pathogenic ancestral strain. (While some strains of E. coli can cause disease, most are harmless or even beneficial. In fact, every person has a billion or so E. coli cells living in their colon.) Every day, including weekends and holidays, each population is propagated by transferring 1% of the previous day’s culture into an equal volume of fresh medium.
The medium is a dilute sugary solution, and glucose is the limiting nutrient. The bacteria easily grow 100-fold in a few hours. However, the glucose then runs out once they’ve grown 100-fold. The cells then sit there until the next day, when we again transfer 1% of each population into fresh medium.
Bacteria reproduce by binary fission: one cell grows and, after it has doubled in size, divides to produce two daughter cells; the two daughter cells then replicate to make four cells; and so on. As it turns out, the 100-fold growth represents about 6 and 2/3 doublings, or generations, each day.
Every 500 generations (75 days), right after the daily transfers are finished, we add a cryoprotectant to the previous day’s cultures. The cryoprotectant helps protect the cells from damage caused by freezing and thawing. We store these samples at –80°C, where they provide a frozen, but viable, fossil record—fossils that can be revived and analyzed far into the future.
These samples allow “time travel” in a scientifically meaningful way. We can, for example, directly compare the current LTEE bacteria with their ancestors. We can even compete the evolved lines against their ancestors, and thereby quantify the results of adaptation by natural selection—the same process that Darwin realized would “fit” organisms to their environment. (Imagine Neanderthals brought back to live among us. How would they fare at chess or football?)
There have been some interruptions along the way, most recently when the LTEE was paused for several months during the COVID-19 pandemic. The frozen samples let us pick up the experiment where we left off. Also, one or more of the 12 populations occasionally fall behind the others, such as when we suspect one line has been accidently “contaminated” by another line. We take great care to avoid these problems, and they happen only rarely; but when they occur, the freezer samples allow us to restart the affected line.
The LTEE environment is kept as simple and constant as possible. The cells have plenty of oxygen; the incubator is kept at 37°C; and there are no competing species or predators in the environment. This environment was not designed to reflect the conditions that E. coli experiences in nature. Rather, the LTEE conditions are simple for the same reason that most experiments in physics and chemistry are conducted in conditions where complicating factors are minimized—namely, to understand what happens in these baseline cases. Nevertheless, even in these simple conditions, complex dynamics have emerged during the LTEE, leading to many unexpected changes and interesting phenomena.
The Goals of the LTEE
The LTEE was designed to explore three sets of broad, conceptual questions. These questions have long interested many scientists, and a lot has been learned from other studies in nature and in the lab. However, the LTEE enables scientists to quantify changes and connect various phenomena with unusual precision.
The first set of questions concerns the dynamics of evolution.
- Is evolution invariably slow and gradual?
- Or are there periods of rapid change and stasis, even in a constant environment?
- How long can fitness continue to improve, and by how much, before some limit is reached?
The second set concerns the repeatability of evolution, especially those changes that are adaptive.
- How repeatable is evolution?
- Will the 12 populations evolve along similar paths?
- Or will they find different phenotypic solutions to their identical environments?
- How does their similarity or divergence depend on which traits are measured?
The third set of questions concerns the coupling of phenotypic and genetic changes.
- Are the rates of phenotypic and genetic evolution tightly coupled over time?
- Or does genetic change continue apace, even after adaptation has slowed or stopped?
- What specific mutations are responsible for the bacteria’s adaptation?
- What are the molecular and physiological changes that make the later generations better adapted to the LTEE environment?
- If and when phenotypes evolve similarly in the replicate populations, does that imply parallel changes at the level of nucleotides, genes, or pathways?
What We’ve Learned from the LTEE
As you can imagine for an experiment that has run for more than 30 years, we’ve learned a lot. Our findings have been reported in over 100 papers published in peer-reviewed scientific journals, as well as in many news stories intended for broader audiences. (Links to the scientific papers and some news stories are provided elsewhere on this website.)
For those wanting non-technical summaries, here are 5 highlights from the LTEE, each in the form of a one-sentence, high-level, take-home message. Each highlight links to a blog post that explains the ideas and supporting evidence in a bit more detail, but still intended for a broad audience.
- Adaptation by Natural Selection: The LTEE provides a simple, compelling demonstration of the process of adaptation by natural selection.
- Endless Adaptations: The bacteria continue to become better and better adapted to the LTEE environment over time, and it appears their fitness may continue to increase indefinitely, albeit at a slower pace.
- Repeatability of Evolution: The LTEE has produced many striking examples of both parallel (repeatable) and divergent changes across the replicate populations.
- Evolution of Novelty: The LTEE provides fascinating cases of the origin and evolution of a new function and complex ecological interactions.
- Changing Mutation Rates: Several LTEE lines have evolved large changes in their spontaneous mutation rates, reflecting a tradeoff between short-term fitness and long-term evolvability.
How Long Will the LTEE Continue?
The LTEE is an experiment that keeps on giving. Between the lapse of time, the inventiveness of the bacteria (even in their simple, confined, little flask worlds), and the many talented students, postdocs, and collaborators who have studied the samples, the LTEE has continued to provide new insights into evolution.
Moreover, new technologies have emerged that weren’t even conceivable when the LTEE began. They have allowed us, for example, to sequence hundreds of genomes from the experiment’s frozen “fossil” record. Who knows what exciting new methods and technologies will be invented and applied to the LTEE in the years, decades, and centuries ahead?
There’s a powerful feedback mechanism at work, as well. These new methods lead to new data, which in turn spark new questions, bring in new people, and lead to new analyses and experiments.
Some years ago, we collected and analyzed data on the fitness trajectories that described the adaptation of the LTEE populations through 50,000 generations. We had previously imagined that the bacteria would reach an asymptote—an upper limit—on their fitness in the simple, static environment of the LTEE. But we discovered instead that the trajectories so far are best described by a simple and elegant equation, called a power law, that has no upper bound. Although this equation predicts that the bacteria will change more slowly as time marches on, it implies that adaptation may continue indefinitely.
I began to wonder what science could achieve if the LTEE ran not just for 50,000 bacterial generations, but for 50,000 scientific generations. Passed from one scientific team to the next every two or three decades for, well, the next million years or so!
I also thought about who should lead the next generation of the LTEE. That person should be young enough to direct the project for years to come, but senior enough to have been promoted and granted tenure at a research-oriented university based on their own scholarly achievements.
And so, I was thrilled when Jeff Barrick accepted my invitation to lead the LTEE into the future! Jeff is an outstanding scientist with expertise in many relevant areas including evolution, microbiology, genomics, bioinformatics, biochemistry, molecular biology, and synthetic biology. He has participated in the LTEE for many years, and he’s made many huge contributions, such as developing and applying the computational pipeline we use to uncover the mutations that distinguish the genomes of the evolved bacteria from the ancestral strain. Moreover, Jeff leads a team of talented students, postdocs, and technicians—a team that not only will sustain the never-ending daily transfers of the LTEE lines, but who will also ask new questions, propose new analyses, and form new collaborations to answer their questions.
Evolve, LTEE, evolve!
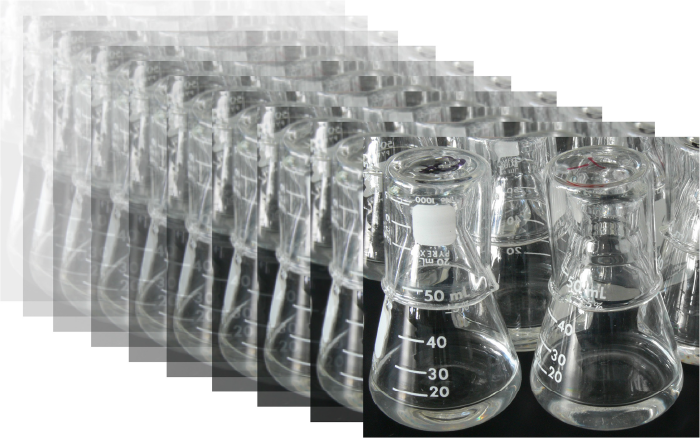